What to know
- Eastern Equine Encephalitis Surveillance and Control Guidelines aim to provide state and local public health officials and mosquito control personnel with up-to-date and accurate information on the surveillance and control of eastern equine encephalitis virus.
- Find information on ecology and epidemiology, surveillance, laboratory diagnosis, integrated vector management, communication, and more.
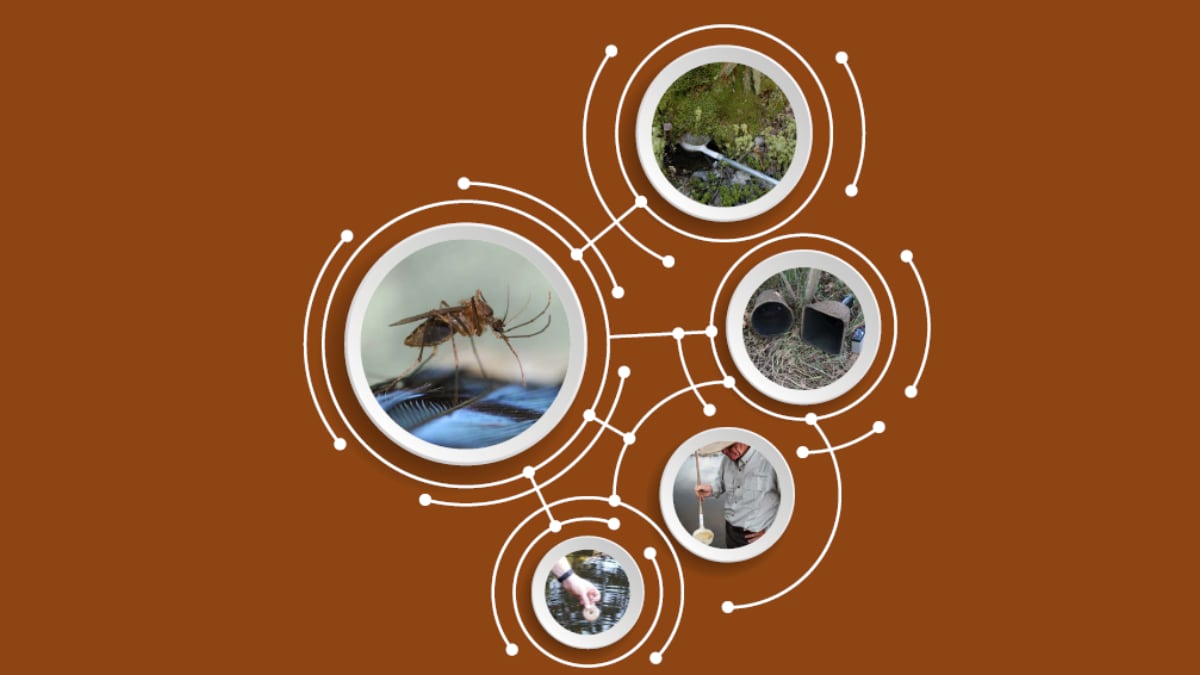
Scope
Comprehensive CDC guidelines for arbovirus surveillance programs in the United States were published in 1993 (CDC 1993). These guidelines detailed best practices for surveillance and control of eastern equine encephalitis (EEE), La Crosse encephalitis (LAC), St. Louis encephalitis (SLE), and western equine encephalitis (WEE). In the several decades since the guidelines were published, EEE has emerged as a vector-borne disease of increasing public health concern due to multiple outbreaks of neuroinvasive disease and the potential for further expansion of EEE into new U.S. regions. Additionally, knowledge about EEE epidemiology and transmission ecology has greatly expanded. The objective of this guidance is to consolidate new knowledge and describe how this can be used to better assess EEE virus activity and mitigate its public health impact. These guidelines are meant for state and local public health officials and mosquito control personnel to aid them in the surveillance and control of EEE.
EEE virus surveillance and control
Eastern equine encephalitis (EEE) virus is an alphavirus endemic to the United States. EEE virus is maintained in the environment mainly by cycling between birds and mosquitoes inhabiting freshwater hardwood swamps (Morris 1988).
Transmission risk is highest in lowland regions where its principal enzootic vector, the ornithophilic mosquito Culiseta melanura readily occurs. Most cases occur in eastern or Gulf Coast states. As with other zoonotic arboviruses, EEE virus persists in a complex ecological web of host species, alternating between mosquito vectors and vertebrate amplification hosts. Habitats that pose a threat to humans and other vertebrates are those that support mosquito species that can serve as vectors and vertebrate hosts that develop viremia of sufficient magnitude to infect mosquitoes (amplification hosts). Reptiles and amphibians have also been implicated in enzootic transmission, particularly in Southern states, perhaps serving as an over-wintering refuge for EEE virus (Graham et al. 2012).
Freshwater swamp and bog habitats are a source ecosystem for EEE virus even in periods of low-level transmission (Miley et al. 2021, Skaff et al. 2021). These freshwater habitats provide pools of water for Cs. melanura larval growth and development and plant nectar for adult mosquito survival. In the Northeast region, EEE virus infections in mosquitoes are correlated with proximity to forested wetland habitat dominated by red maple (Acer rubrum) and Atlantic white cedar (Chamaecyparis thyoides). The dominant trees shift to bald cypress (Taxodium distichum) and water tupelo (Nyssa aquatica) in the southeast bottomlands. In the Great Lakes region, Cs. melanura favor sphagnum bogs for oviposition and larval development. Primary buttressed trees in these habitats include black spruce, red spruce, yellow birch, and tupelo.
In the late summer, bird populations disperse after breeding, causing declines in easily available avian blood meals. Swamp-inhabiting mosquitoes may then seek blood outside of the swamp habitats, increasing risk that the virus will spill over to infect other vertebrates and secondary vectors. Surveillance for EEE virus-infected hosts routinely detects vertebrate and vector infections within the swamp habitat, and during epizootic and epidemic transmission outside their primary habitat. Some secondary vectors act as bridge vectors, feeding on birds and mammals and transmitting virus from enzootic to epizootic and epidemic hosts. Once spillover occurs, surveillance can detect EEE virus infections in other potential vector mosquito species.
Vectors suspected of transmitting EEE virus to horses and humans vary geographically. Culiseta melanura can act as both an enzootic and epidemic vector (Armstrong and Andreadis 2010). Primary bridge vectors include Coquillettidia perturbans, Aedes canadensis, Ae. sollicitans, and Culex salinarius in the Northeast and Mid-Atlantic regions (Armstrong and Andreadis 2010; Armstrong and Andreadis 2022; Crans 1977), Cq. perturbans, Ae. canadensis, Cx. salinarius, and Cx. erraticus in the Southeast (Burkett-Cadena et al. 2022), and Cq. perturbans in the Midwest (Nasci et al. 1993). Bridge vectors are competent to transmit virus and infected mosquitoes of these species are frequently detected during epizootic and epidemic periods. Horses and humans are considered dead-end hosts because they do not develop high enough levels of EEE virus in their blood to infect feeding mosquitoes.
The first human EEE disease cases were recognized during a 1938 outbreak in southeastern Massachusetts (Feemster 1938). Subsequent outbreaks were then reported in New Jersey during the 1950s (Goldfield and Sussman 1968). Since then, EEE cases have occurred sporadically and in small clusters, most around freshwater hardwood swamps in the Atlantic and Gulf Coast states and the Great Lakes region (Goldfield and Sussman 1968; Przelomski 1988; CDC 2006). Human infections typically occur during summer months, with >80% of reported human cases having an illness onset during July through September (Lindsey et al. 2018). During 2003–2018, an average of eight EEE disease cases were reported annually in the United States (range = 4–21 cases/year) (Lindsey 2018; CDC 2021). However, in 2019, 38 cases were reported nationally (Vahey et al. 2021). The reasons for this increase are unknown but are likely related to several factors, including weather, abundance of birds and mosquitoes that can transmit the virus, human behavior, and clinical awareness and diagnostic testing practices.
Routes of Transmission
EEE virus is maintained in a cycle between Culiseta melanura mosquitoes and avian hosts in freshwater hardwood swamps. Culiseta melanura is not considered to be an important vector of EEE virus to people because it feeds almost exclusively on birds. Transmission to people requires another mosquito species to create a bridge between infected birds and uninfected mammals, such as people or horses (Morris 1988). Most of the bridge species are within the Aedes, Coquillettidia, and Culex genera. EEE virus has been documented to be transmitted through organ transplantation with one organ donor transmitting the infection to three organ transplant recipients (Pouch et al. 2019). Although not documented, EEE virus likely can be transmitted from person-to-person through blood transfusions.
Clinical Presentation and Evaluation
Most persons infected with EEE virus have no apparent illness (Davis et al. 2008; Calisher 1994). Among those who develop symptoms, the incubation period typically ranges from 4 to 10 days but can be several weeks in people who are immunocompromised (CDC 2021; Sherwood and Oliver 2013).
Symptomatic infection is characterized by fever, chills, malaise, arthralgia, and myalgia (Calisher 1994). Most people recover completely in 1 to 2 weeks unless central nervous system involvement is present. Less than 5% of infected individuals develop meningitis or encephalitis (Morris 1988; Goldfield et al.1968). In infants, neurologic disease often occurs soon after onset; in older children and adults, encephalitis may occur after several days of systemic illness. Signs and symptoms in patients with neuroinvasive disease include headache, confusion, focal neurologic deficits, meningismus, seizures, or coma (Feemster 1938; Przelomski et al.1988; Deresiewicz et al. 1997; Clarke 1961; Letson et al. 1993; Ayers and Feemster 1949). Cerebrospinal fluid (CSF) findings include an initial neutrophil-predominant pleocytosis, shifting to a lymphocyte-predominance, and elevated protein levels; glucose levels are normal (Przelomski et al. 1988; Deresiewicz et al. 1997; Silverman et al. 2013). Neuroimaging shows brain lesions consistent with encephalitis, including neuronal destruction and vasculitis in the cortex, midbrain, and brain stem (Przelomski et al. 1988; Silverman et al. 2013). Magnetic resonance imaging using T2-weighted images often show areas of increased signal in basal ganglia and thalami (Deresiewicz et al. 1997).
Persons aged >50 and <15 years seem to be at greatest risk for developing severe disease when infected with EEE virus. EEE neuroinvasive disease is estimated to have a 30% case fatality rate and results in neurologic sequelae in >50% of survivors (Feemster 1938; Goldfield and Sussman 1968; Deresiewicz et al. 1997; Letson et al. 1993; Ayers and Feemster 1949; Silverman et al. 2013; Gaensbauer et al. 2014). Death typically occurs 2 to 10 days after symptom onset but can occur much later. The neurologic sequelae can range from mild brain dysfunction to severe intellectual impairment, personality disorders, seizures, paralysis, and cranial nerve dysfunction. Many patients with severe sequelae require long-term care and die within a few years.
EEE virus disease should be considered in any person with a febrile or acute neurologic illness with a potential for recent exposure to mosquitoes, organ transplantation, or potentially blood transfusion, particularly during the summer months in areas where virus activity has been reported. In addition to other more common causes of encephalitis and aseptic meningitis (e.g., herpes simplex virus and enteroviruses), other arthropod-borne viruses (e.g., West Nile, La Crosse, St Louis encephalitis, and Powassan viruses) should also be considered in the differential diagnosis of suspected EEE.
Arboviral surveillance consists of two distinct, but complementary activities. Epidemiological surveillance measures human disease to quantify disease burden, detects early signs of an outbreak, and identifies information needed for timely responses, including seasonal, geographic, and demographic patterns in human morbidity and mortality. In addition to monitoring disease burden and distribution, epidemiological surveillance has been instrumental in characterizing clinical disease presentation and disease outcome, as well as identifying high-risk populations and human factors associated with serious disease. Epidemiological surveillance has also detected and quantified alternative routes of transmission to humans, such as contaminated blood donations and organ transplantation. Environmental surveillance monitors local mosquito populations, virus activity in vectors and non-human vertebrate hosts, and other relevant environmental parameters to predict human risk and prevent outbreaks of arboviral disease in humans. Epidemiological and environmental surveillance for arboviruses is facilitated by ArboNET, the national arbovirus surveillance system. ArboNET was developed in 2000 as a comprehensive surveillance data capture platform to monitor West Nile virus (WNV) infections in humans, mosquitoes, birds, and other animals. This comprehensive approach was essential to tracking the progression of WNV as it spread and became established across the United States, and it remains a significant source of data on the epidemiology and ecology of WNV. Since 2003, ArboNET has also collected data on other domestic and exotic arboviruses of public health significance.
In the absence of effective human vaccines for most domestic arboviruses, preventing arboviral disease in humans primarily depends on measures to keep infected vectors from biting people. A principal objective of environmental surveillance is to quantify the intensity of virus transmission in a region and provide a predictive index of human infection risk. This risk prediction, along with information about the local conditions and habitats, and virus-vector interactions that impact vector abundance and infection, can be used to inform an Integrated Vector Management (IVM) program and decisions about implementing interventions to control mosquitoes and to subsequently prevent disease.
Though epidemiological surveillance is essential for understanding arboviral disease burden, utilizing human case surveillance by itself is insufficient for predicting outbreaks. Outbreaks can develop quickly, with most human cases occurring over a few weeks during the peak of transmission. The time from human infection to onset of symptoms to diagnosis and reporting can be several weeks or longer. As a result, human case reports typically lag well behind the transmission from vectors that initiated the infection. Cases in non-human vertebrate hosts are often the first indicator of local arboviral activity. Comparing infection prevalence in vectors and non-human vertebrate cases with historical environmental and epidemiological data can help identify conditions associated with human risk 2 to 4 weeks before human disease onset. This provides additional lead time for critical vector control interventions and public education programs to be put in place. The following sections describe the elements of epidemiological and environmental arboviral surveillance and how they may be used to monitor and predict risk and to trigger interventions.
Passive Surveillance and Case Investigation
Arboviral diseases are nationally notifiable conditions, and many are explicitly reportable in U.S. states and territories. Most disease cases are reported to public health authorities from public health or commercial laboratories; healthcare providers can also directly submit reports of suspected cases. State and local health departments are responsible for ensuring that reported human disease cases meet the national case definitions. The most recent case definitions for arboviral diseases can be located on the CDC Nationally Notifiable Disease Surveillance System website (https://ndc.services.cdc.gov/conditions/arboviral-diseases-neuroinvasive-and-non-neuroinvasive/). For some arboviruses (e.g., West nile virus (WNV)) presumptive viremic donors are identified through universal screening of the blood supply; case definitions and reporting practices for viremic donors vary by jurisdiction and blood services agency. All identified human disease cases and presumptive viremic blood donors should be investigated promptly. Jurisdictions may choose to interview the patient's healthcare provider, the patient, or both depending on information needs and resources. Whenever possible, the following information should be gathered
- Basic demographic information (e.g., age, sex, race/ethnicity, state, county of residence)
- Clinical syndrome (e.g., asymptomatic blood donor, uncomplicated fever, meningitis, encephalitis, acute flaccid paralysis)
- Illness onset date and/or date of blood donation
- If the patient was hospitalized and if he/she survived or died
- Travel history in the 4 weeks prior to onset
- If the patient was an organ donor or a transplant recipient in the 4 weeks prior to onset
- If the patient was a blood donor or blood transfusion recipient in the 4 weeks prior to onset
- If the patient was pregnant at illness onset
- If the patient is an infant, was he/she breastfed before illness onset
If the patient donated blood, tissues, or organs in the 4 weeks prior to illness onset, immediately inform the blood or tissue bank and appropriate public health authorities. Similarly, any infections temporally associated with blood transfusion or organ transplantation should be reported. Prompt reporting of these cases will facilitate the identification and quarantine of any remaining infected products and the identification of any other exposed recipients so they may be managed appropriately.
Passive surveillance systems are dependent on clinicians considering the diagnosis of an arboviral disease and obtaining the appropriate diagnostic test and reporting of laboratory-confirmed cases to public health authorities. Because of incomplete diagnosis and reporting, the incidence of arboviral diseases is underestimated. Where applicable, reported neuroinvasive disease cases are considered the most accurate indicator of activity in humans because of the substantial associated morbidity. In contrast, reported cases of non-neuroinvasive disease are more likely to be affected by disease awareness and healthcare-seeking behavior in different communities and by the availability and specificity of laboratory tests performed.
Enhanced Surveillance Activities
Enhanced surveillance for human arboviral disease cases should be considered when environmental or human surveillance suggests that an outbreak is suspected or anticipated. Educating healthcare providers and infection control practitioners about the need for arbovirus testing and reporting of all suspected cases could increase the sensitivity of the surveillance system. This might be accomplished by distributing print materials, participating in local hospital meetings and grand rounds, and providing lectures/seminars. Public health agencies should also work to establish guidelines and protocols with local blood collection agencies for reporting viremic blood donors. At the end of the year, an active review of medical records and laboratory results from local hospitals and associated commercial laboratories should be conducted to identify any previously unreported cases. In addition, an active review of appropriate records from blood collection agencies could be conducted to identify any positive donors that were not reported.
Vector-based Surveillance
Vector surveillance is an integral component of an Integrated Vector Management (IVM) program and is the primary tool for quantifying virus transmission and human risk. The principal functions of a mosquito-based surveillance program are to:
- Collect data on mosquito population abundance and virus infection rates in those populations
- Provide indicators of the threat of human infection and identify geographic areas of high risk
- Support decisions regarding the need for and timing of intervention activities (e.g., enhanced vector surveillance and control, use of new technologies and public education programs)
- Monitor the effectiveness of vector control methods, including susceptibility of target mosquitoes to control methods used
Mosquito-based arboviral monitoring complements disease surveillance programs by contributing timely results and data for action. Programs maintaining in-house laboratories may be able to process mosquito samples daily, giving results within a few days. Data on vector species community composition, relative abundance, and infection rates allow programs to rapidly compute infection indices, assess risk, and respond. Maintaining mosquito surveillance over the long-term provides a baseline of historical data to evaluate risk and guide mosquito control operations. However, the utility of mosquito-based surveillance depends both on the type and quality of data collected (e.g., number and type of traps, timing and frequency of sampling, testing procedures) and consistent effort across transmission seasons to link surveillance indices with human risk.
There are three main categories of vector surveillance: larval, adult, and transmission activity. Together, this information is used to determine where and when control efforts should be implemented. Larval surveillance involves sampling a wide range of aquatic habitats to identify the sources of vector mosquitoes and evaluating larval control measures applied. For adult mosquitoes, regular (e.g., monthly, weekly) sampling is done at fixed sites throughout the community that are representative of the habitat types present in the area. Adult mosquitoes are collected using a variety of trapping techniques, including traps for host-seeking, resting, or gravid (carrying eggs) mosquitoes seeking a place to lay eggs (oviposition site). Adult surveillance can also be used to evaluate control activities pre- and post-treatment. Transmission activity surveillance provides information on the level of infected mosquitoes in an area.
Specimen Collection and Traps
Mosquito species involved in enzootic or epidemic transmission are readily captured in CDC light traps (with or without CO2) and New Jersey light traps. For best results, the traps need to be placed in well-protected sites with very limited wind movement. Resting boxes may be used to increase the chances of capturing infected mosquitoes, and the CDC battery powered resting box traps can increase the number of mosquitoes captured, as well as improve consistency and ease of sampling (Panella et al. 2011). The resting populations can also be collected using backpack aspirators (e.g., modified CDC backpack aspirator https://www.johnwhock.com/products/aspirators/modified-cdc-backpack-aspirator/, or the lightweight battery-powered aspirator [Nasci 1981]) to remove mosquitoes from natural harborages or artificial resting structures (e.g., wooden resting boxes, red boxes, fiber pots, and other similar containers (Holderman et al. 2018)).
Specimen Handling and Processing
Because mosquito-based surveillance relies on identifying virus in the collected mosquitoes through detection of viral proteins, viral RNA, or live virus (see Laboratory Diagnosis and Testing section), specimens should be handled in a way that minimizes exposure to conditions (e.g., heat, successive freeze-thaw cycles) that would degrade the virus. Optimally, a cold chain should be maintained from the time mosquitoes are removed from the traps to the time they are delivered to the processing laboratory. Mosquitoes can be transported from the field in a cooler with cold packs or on dry ice, and then placed on a chill-table, if available, during sorting, identification, and pooling. Usually only female mosquitoes are tested in routine arboviral surveillance programs. If virus screening is not done immediately after mosquito identification and pooling, the pooled samples should be stored frozen (e.g., -70°C) or at temperatures below freezing for short-term storage. Although the lack of a cold chain might impact the ability to culture the virus, it does not appear to reduce the ability to detect viral RNA by reverse transcription-polymerase chain reaction (RT-PCR) (Turell et al. 2002).
Vector-based Surveillance Indicators
Data derived from mosquito surveillance include estimates of mosquito species abundance and infection rate in those mosquito populations. The indices derived from those data vary in information content, ability to be compared over time and space, and association with transmission levels and levels of human risk. Five indicators that have commonly been used include: vector abundance, number of positive pools, percent of pools positive, infection rate, and vector index (Table 1).
Vector abundance provides a measure of the relative number of mosquitoes in an area during a particular sampling period. It is the total number of mosquitoes of a particular species collected, divided by the number of trapping nights during a specified sampling period, and is expressed as the number/trap night. Risk assessments often consider mosquito abundance because high mosquito densities can be associated with arboviral disease outbreaks (Olson et al. 1979; Eldridge 2004). For example, during a WNV outbreak in Maricopa County, AZ in 2010, Culex quinquefasciatus densities were higher in outbreak compared to non-outbreak areas (Godsey et al. 2012; Colborn et al. 2013). High Culiseta melanura and Coquillettidia perturbans abundance has also been associated with elevated eastern equine encephalitis (EEE) virus activity. However, high mosquito abundance can occur in the absence of virus, and outbreaks can occur when abundance is low, but the vector infection rate is high. Vector abundance measures are used for planning IVM and monitoring the outcomes of mosquito control. Number of traps, their distribution, and the timing of sample collection should be sufficient to obtain spatially and temporally representative data.
Number of positive pools is the total of the number of arbovirus positive mosquito pools detected in a given surveillance location and period. These may be a tally of the total positive pools separated by species or for all species tested. This indicator provides evidence of arboviral activity, particularly during field investigations and outbreak response, but is not recommended as a stand-alone indicator. Instead, data can be used to produce more informative indices (i.e., infection rate and vector index).
Percent of pools positive is calculated by the number of positive pools divided by the total number of pools tested, as a percentage. It provides data that can be used to compare activity over time and place. However, the comparative value is limited unless the number of pools tested is large and the number of mosquitoes per pool remains constant. As with the number of positive pools index, these data can be used for calculation of the, often more informative, infection rate and vector index.
The infection rate in a sampled vector population estimates the true infection prevalence of infected mosquitoes in the population and is a good indicator of human risk. It provides a useful, quantitative basis for comparison, allowing evaluation of changes in population infection prevalence over time and space. Infection rate indices have been used successfully to link infection rates with human risk (Bell et al. 2005). When computing infection rate indices, variable pool numbers and pool sizes can be used, while retaining comparability, but larger sample sizes improve precision. Two methods are commonly used to calculate infection rate:
- Minimum infection rate (MIR) for a given mosquito species is the number of positive pools divided by the total number of mosquitoes tested. Use of the MIR assumes that infection rates are low and that only one mosquito is positive in a positive pool.
- Maximum likelihood estimate (MLE) corrected for bias is the preferred method, particularly during outbreaks. MLE-associated estimates are based on binomial probability models for pooled data and do not assume only one positive mosquito per positive pool. Bias-corrected MLEs provide more accurate estimates than the standard MLE (Biggerstaff 2008; Hepworth and Biggerstaff, 2017, 2021) and are more accurate than the MIR (Gu et al. 2008; Biggerstaff 2008). MLE-based estimates are computed from straightforward formulas when there is only one pool size, but computer iterative methods are needed when pool sizes differ. Both an R package and a Microsoft Excel Add-in are available to compute infection rate estimates from pooled data.
While the MLE-based estimates and the MIR are similar when infection rates are low, the assumption underlying the use of the MIR is untenable as the true infection rate increases, the MIR is less accurate than bias-corrected MLEs, and in any case confidence intervals based on the MIR have been shown to be poor (e.g., Biggerstaff 2008).
The Vector Index (VI) estimates the relative abundance of infected mosquitoes in an area and incorporates into a single index information on presence, relative abundance, and infection rates of individual species (Gujaral et al. 2007; Bolling et al. 2009; Jones et al. 2011). The VI is calculated by multiplying the average number of mosquitoes collected per trap night by the infection rate. VI is expressed as the average number of infected mosquitoes collected per trap night in the area during the sampling period. In areas with multiple vector species, a VI is calculated for each species, then individual VIs are summed to give a combined estimate of infected vector relative abundance.
Increases in VI reflect increased risk of human disease and serves as a more reliable prediction measure than vector abundance or infection rate alone (Bolling et al. 2009; Jones et al. 2011; Kwan et al. 2012; Colborn et al. 2013). As with other surveillance indicators, the accuracy of the VI depends on the number of trap nights used to estimate abundance and the number of specimens tested to estimate infection rate.
Use of Vector-based Surveillance Indicators. Mosquito-based surveillance indicators have two important roles in arboviral surveillance and response programs. First, they can provide quantifiable thresholds for proactive vector control efforts and public health messaging. By identifying thresholds for vector abundance and infection rates that are below levels associated with disease outbreaks, IVM programs can institute proactive measures to maintain mosquito populations at levels below which virus transmission would be likely. Second, if thresholds related to outbreak levels of transmission can be identified, surveillance can help determine when proactive measures were insufficient to dampen virus amplification and more aggressive measures are needed, such as expanded mosquito control measures and public messaging.
Table 1. Summary of Mosquito-Based Surveillance Indicators
ArboNET, the national arboviral surveillance system, was developed by CDC and state health departments in 2000 in response to the emergence of WNV in 1999. Since its development, ArboNET has expanded to include many other arboviruses of public health importance. ArboNET is an electronic surveillance system administered by CDC's Division of Vector-Borne Diseases (DVBD). Human arboviral disease data are reported from all states, territories, and associated states. In addition to human disease cases, ArboNET maintains data on arboviral infections among human viremic blood donors, non-human mammals, sentinel animals, dead birds, and mosquitoes.
Data Collected. Variables collected for human disease cases include patient age, sex, race, and county and state of residence; date of illness onset; case status (i.e., confirmed, probable, suspected, or not a case); clinical syndrome (e.g., encephalitis, meningitis, or uncomplicated fever); whether illness resulted in hospitalization; and whether the illness was fatal. Cases reported as encephalitis (including meningoencephalitis), meningitis, or acute flaccid paralysis are collectively referred to as neuroinvasive disease; others are considered non-neuroinvasive disease. Acute flaccid paralysis can occur with or without encephalitis or meningitis. Information regarding potential non-mosquito-borne transmission (e.g., blood transfusion or organ transplant recipient, breast-fed infant, or laboratory worker) and recent donation of blood or solid organs should be reported if applicable. Clinical symptoms and diagnostic testing data can also be reported.
Blood donors identified as presumptively viremic by nucleic acid amplification test (NAAT) screening of the donation by a blood collection agency are also reported to ArboNET. Case definitions have been developed for the purposes of national surveillance. The date of blood donation is reported in addition to the variables routinely reported for disease cases.
Arboviral disease in non-human mammals (primarily horses) and infections in trapped mosquitoes, dead birds, and sentinel animals (primarily chickens) are also reported to ArboNET. Variables collected for non-human infections include species, state and county, and date of specimen collection or symptom onset. Until 2023, the total number of mosquitoes or birds tested weekly also could be reported by county and species.
Detailed descriptions of all variables collected by ArboNET and instructions for reporting are included in the ArboNET User Guide, which can be requested from DVBD by phone (970-261-6400) or email ([email protected]).
Data Transmission. Jurisdictions can transmit data to ArboNET using one or more of four methods supported by DVBD: 1) jurisdictions that have a commercially- or state-developed electronic surveillance system can upload records from their system using an Extensible Markup Language (XML) message; 2) jurisdictions can upload records from a Microsoft Access database provided by CDC DVBD using an XML message; 3) jurisdictions may enter records manually using a CDC website (https://csams.cdc.gov/arbonet); or 4) jurisdictions can report cases using an HL-7 message via the CDC National Electronic Disease Surveillance System (NEDSS), and DVBD will download records directly from NEDSS to ArboNET. ArboNET data are maintained in a Microsoft Structured Query Language (SQL) Server database inside CDC's firewall (note this is likely to change soon and potentially during the 2023 season). Users can access data via a password-protected website but are limited to viewing data only from their own jurisdiction. The ArboNET website and database are maintained by CDC information technology staff and are backed up nightly.
Dissemination of ArboNET Data. CDC epidemiologists periodically review and analyze ArboNET surveillance data and disseminate results to stakeholders via direct communication, briefs in Morbidity and Mortality Weekly Reports and Epi-X, comprehensive annual summary reports, and DVBD's website. CDC also produces maps of domestic and exotic arboviral activity, which are then posted on CDC's disease-specific websites. Surveillance reports are typically updated biweekly during the transmission season and monthly during the off-season. A final report is usually released in the spring of the following year. CDC provides limited-use ArboNET data sets to the general public by formal request. Data release guidelines have been updated to be consistent with those developed by CDC and the Council of State and Territorial Epidemiologists.
Limitations of ArboNET Data. Human surveillance for arboviral disease is largely passive and relies on the receipt of information from physicians, laboratories, and other reporting sources by state health departments. For viruses that can cause neuroinvasive disease, neuroinvasive disease cases are likely to be consistently reported because of the substantial morbidity associated with this clinical syndrome. In comparison, non-neuroinvasive disease cases are inconsistently reported because of a less severe spectrum of illness, geographic differences in disease awareness and healthcare-seeking behavior, and variable capacity for laboratory testing. Surveillance data for fever cases associated with neuroinvasive arboviruses should be interpreted with caution and generally should not be used to make comparisons between geographic areas or over time. Accordingly, ratios of reported neuroinvasive disease cases to non-neuroinvasive disease cases should not be interpreted as a measure of virulence in an area.
ArboNET does not routinely collect information regarding clinical signs and symptoms or diagnostic laboratory test results. Therefore, misclassification of the various syndromes caused by arboviruses cannot be detected. In addition, ArboNET does not routinely collect information regarding the specific laboratory methods used to confirm each case. Although serologic assays are relatively specific, false-positive results and cross-reactions occur between related viruses (e.g., flavivirus, such as West Nile, SLE, and dengue viruses, or California serogroup viruses, such as La Crosse and Jamestown Canyon viruses). Positive IgM antibody results should be confirmed by additional tests, especially plaque-reduction neutralization test (PRNT). However, such confirmatory testing often is not performed. While the electronic mechanisms for data transmission allow for rapid case reporting, the inclusion of both clinical and laboratory criteria in the surveillance case definition creates delays between the occurrence of cases and their reporting. Provisional data are disseminated to allow for monitoring of regional and national epidemiology during the arboviral transmission season. However, these reports generally lag several weeks behind the occurrence of the cases comprising them, and the data may change substantially before they are finalized. For this reason, provisional data from the current transmission season should not be combined with or compared to provisional or final data from previous years.
The collection and reporting of non-human surveillance data are highly variable among states (and even between regions within states) and changes from year to year. Because of this variability, non-human surveillance data should not be used to compare arboviral activity between geographic areas or over time.
For more information about ArboNET, please contact the Division of Vector-Borne Diseases by phone: 970-261-6400 or email: [email protected].
Laboratory Diagnosis of Human Arboviral Diseases
Laboratory testing for evidence of arboviral diseases typically involves serologic and molecular testing. For several viruses where humans are an amplification host, molecular testing is more specific and can be used to confirm the diagnosis in the first week of illness. For viruses that typically are neuroinvasive, serology is more likely to be used to determine if someone was recently infected.
In most patients, infection with an arbovirus that can cause encephalitis is clinically inapparent or causes a nonspecific viral syndrome. Numerous pathogens cause encephalitis, aseptic meningitis, and febrile disease with similar clinical symptoms and presentations and should be considered in the differential diagnosis. Definitive diagnosis can only be made by laboratory testing using specific reagents. Selection of diagnostic test procedures should take into consideration patient factors (e.g., age, immune status, vaccination history), timing of infection, the range of pathogens in the differential diagnosis, the criteria for classifying a case as confirmed or probable, as well as the capability of the primary and confirming diagnostic laboratories.
Appropriate selection of diagnostic procedures and accurate interpretation of findings requires information describing the patient and the diagnostic specimen. For human specimens, the following data must accompany sera, CSF or tissue specimens for results to be properly interpreted and reported: 1) symptom onset date (when known); 2) date of sample collection; 3) unusual immunological status of patient (e.g., immunosuppression); 4) state and county of residence; 5) travel history (especially in flavivirus-endemic areas); 6) history of prior vaccination (e.g., yellow fever, Japanese encephalitis, or tick-borne encephalitis viruses); and 7) brief clinical summary including clinical diagnosis (e.g., encephalitis, aseptic meningitis). Minimally, onset and sample collection dates are required to perform and interpret initial screening tests. The remaining information is required to evaluate any test results from initial screening. If possible, a convalescent serum sample taken at least 14 days following the acute sample should be obtained to enable confirmation by serological testing.
Human Diagnostic Testing
Eastern equine encephalitis (EEE) virus is a HHS Select Agent, and therefore, subject to strict regulations regarding its possession and use. Those intending to conduct EEE virus testing must be familiar with the complete information and specific guidance found at the Federal Select Agent Program website before conducting EEE virus testing.
Briefly, samples determined to be positive for EEE virus must be documented and reported to the Federal Select Agent Program via Form 4 (https://www.selectagents.gov/form4.html) within 7 calendar days of identification, and, if not diagnosed at a registered entity, they must then be transferred to a registered Select Agent facility or destroyed with documentation.
Serology
The front-line diagnostic assay for laboratory diagnosis of human EEE virus infection is the IgM antibody assay. Commercially available immunofluorescence assay (IFA) kits to detect IgM or IgG antibodies are often used in public health and other laboratories the United States. In addition, IgM and IgG assays developed at CDC are available in both ELISA and microsphere (IgM) immunoassay (MIA; Basile et al. 2013) formats; protocols and limited supplies of reagents are available from CDC's DVBD Diagnostic Laboratory. CDC will provide positive controls and limited reagents considering commercial sources are available to state public health labs.
Because the IgM and IgG assays can be positive due to non-specific reactivity or rarely cross-reactivity (e.g., EEE virus is the only virus in the EEE antigenic complex in the United States, but low-level cross-reactivity might occur with other alphaviruses), they should be viewed as a presumptive positive. For a case to be considered confirmed, serum samples that are antibody-positive on initial testing should be evaluated by a more specific assay. Currently, the plaque reduction neutralization test (PRNT) is recommended for confirming IgM serological results. Although EEE virus is a rare cause of arboviral encephalitis in the United States, several other arboviral encephalitides are present in the United States and in other regions of the world. Specimens submitted for EEE virus testing should also be tested by ELISA and PRNT against other arboviruses known to be active or present in the area or in the region to where the patient traveled.
Virus Detection Assays
Numerous procedures have been developed for detecting viable EEE virus, EEE virus antigen, or EEE virus RNA in human diagnostic samples, many of which have been adapted to detecting EEE virus in other vertebrates and in mosquito samples. These procedures vary in their sensitivity, specificity, and time required to conduct the test. Among the most sensitive procedures for detecting EEE virus in samples are those using RT-PCR to detect EEE virus RNA in human CSF, serum, and other tissues. Real-time RT-PCR, standard RT-PCR, and nucleic acid sequence-based amplification (NASBA) amplification methods have been developed and validated for specific human diagnostic applications (Lambert et al. 2003); however, no commercially-produced or FDA-approved molecular EEE virus diagnostic tests are available.
EEE virus presence can be demonstrated by isolation of viable virus from samples taken from clinically ill patients. Appropriate samples include CSF, serum samples obtained very early in infection, and brain tissue taken at biopsy or postmortem. Virus isolation should be performed in known susceptible mammalian (e.g., Vero) or mosquito cell lines (e.g., C6/36). Mosquito origin cells may not show obvious cytopathic effect and must be screened by immunofluorescence or RT-PCR. Confirmation of virus isolate identity can be accomplished by indirect immunofluorescence assay (IFA) using virus-specific monoclonal antibodies (MAbs) or nucleic acid detection (e.g., RT-PCR, real-time RT-PCR, or sequencing). The IFA using well-defined murine MAbs is an efficient, economical, and rapid method to identify alphaviruses isolated in cell culture. Incorporating MAbs specific for other arboviruses known to circulate in various regions will increase the rapid diagnostic capacities of state and local laboratories. Nucleic acid detection methods include real-time and standard RT-PCR methods.
Immunohistochemistry (IHC) using virus-specific MAbs on tissue has been useful in identifying both human and veterinary cases of EEE virus infection. In suspected fatal cases, IHC should be performed on formalin fixed autopsy, biopsy, and necropsy material, ideally collected from multiple anatomic regions of the brain, including the brainstem, midbrain, and cortex.
Resources for Human Diagnostic Laboratories
Clinical Laboratory Improvements Amendments (CLIA) certification: To maintain certification, CLIA recommendations for performing and interpreting human diagnostic tests should be followed. Laboratories performing arboviral serology or RNA-detection testing are invited to participate in the annual proficiency testing that is available from CDC's Division of Vector-Borne Diseases (DVBD) in Fort Collins, CO. To obtain additional information about the proficiency testing program and about training in arbovirus diagnostic procedures, contact the DVBD by phone: 970-261-6400 or email: [email protected].
Biocontainment: Containment specifications are available in the CDC/National Institutes of Health publication Biosafety in Microbiological and Biomedical Laboratories (BMBL 6).
Shipping of diagnostic samples and agents. Shipping and transport of clinical specimens should follow current International Air Transport Association (IATA) and Department of Commerce recommendations. For more information, visit the IATA dangerous goods Web site at: http://www.iata.org/publications/dgr/Pages/index.aspx, and the USDA Animal and Plant Health. Inspection Service (APHIS), National Center for Imports and Exports website: https://www.aphis.usda.gov/aphis/ourfocus/importexport.
Laboratory testing of vectors
Identification and Pooling
Mosquitoes should be identified to species or lowest taxonomic unit. Specimens are placed into pools of 50 specimens or less based on species, sex, location, trap-type, and date of collection. Larger pool sizes can be used in some assays with loss of sensitivity (Sutherland and Nasci 2007). If resources are limited, testing of mosquitoes for surveillance purposes can be limited to the primary vector species.
Homogenizing and Centrifugation
After adding an appropriate homogenization buffer (cell culture medium, viral transport medium, PBS), mosquito pools can be macerated or ground by a variety of techniques including mortar and pestle, vortexing sealed tubes containing one or more copper clad BBs, or by use of tissue homogenizing apparatuses that are commercially available. After grinding, samples are centrifuged, and an aliquot is removed for testing. Because mosquito pools may contain arboviruses and other pathogenic viruses which may be aerosolized during processing, laboratory staff should take appropriate safety precautions including use of a Class II Type A biological safety cabinet, appropriate personal protective equipment (PPE) and biosafety practices.
Virus Detection
EEE virus is an HHS Select Agent, and therefore, subject to strict regulations regarding its possession and use. Those intending to conduct EEE virus testing must be familiar with the complete information and specific guidance found at the Federal Select Agent Program website before conducting EEE virus testing.
Briefly, samples determined to be positive for EEE virus must be documented and reported to the Federal Select Agent Program via Form 4 (https://www.selectagents.gov/form4.html) within 7 calendar days of identification, and, if not diagnosed at a registered entity, they much then be transferred to a registered Select Agent facility or destroyed.
Multiple diagnostic methods can be used to identify EEE virus in mosquitoes including virus isolation, molecular assays, or immunoassays. Virus isolation in Vero cell culture (Armstrong et al. 2011) remains the standard for confirmation of positive pools but is time consuming and requires specialized laboratory facilities. For virus isolation, mosquito pool homogenates are added to Vero cell cultures, monitored for cytopathic effect, and identified using appropriate diagnostic assays. Aliquots are stored at -70°C to retain virus viability for future testing. Vero cell culture has an additional benefit of detecting other viruses in the mosquitoes, a feature lost using test procedures that target virus-specific nucleotide sequence or proteins (Andreadis et al. 1998; Andreadis et al. 2004). Molecular assays detect viral RNA or nucleic acids in mosquito pools quickly, with high sensitivity and specificity, and do not require cold chain or high levels of biocontainment (Lanciotti et al. 2000; Callahan et al. 2001; Lambert et al. 2003; Armstrong et al. 2012). Methods include rapid molecular assays, RT-PCR, and other nucleic acid amplification tests (e.g., multi-target RT-PCR). Assays may use different primer sets for detection and confirmation of virus in mosquito pools (Lambert et al. 2003). Antigen detection assays are less sensitive than molecular assays, but for some arboviruses (e.g., WNV) can provide valuable infection rate data when employed consistently in a mosquito surveillance program. For additional details see: Human Diagnostic Detection and Annex 1 Real Time RT-PCR Protocol for Mosquito Pools.
Laboratory Testing of Non-human Vertebrates
The choice of laboratory diagnostic tests depends on the needs, approach, and surveillance capability of a given health agency. Tests include antibody-capture ELISA, complement fixation (CF), hemagglutination inhibition (HI), and plaque reduction neutralization test (PRNT). However, few reagents are commercially available for domestic or wildlife IgM antibodies for antibody capture ELISAs. Many public health laboratories will therefore use PRNT because these are not dependent on species specific antibodies.
Serology
The same serologic techniques applied to clinically ill animals may also be used for healthy subjects for vertebrate serosurveys or for healthy sentinel animals serially-sampled as sentinels. As with human diagnostic samples, serologic results from non-human vertebrates must be interpreted with caution due to potential cross-reactivity. Cross reactions may occur between EEE and WEE antibodies in the CF and HI tests. Vaccination for EEE can also cause positive PRNT, HI, CF, and possibly IgM test results.
Specimens from horses and other domestic animals can be tested through diagnostic laboratories including U.S. Department of Agriculture's (USDA) Animal and Plant Health Inspection Service's (APHIS) National Veterinary Services Laboratory (NVSL) in Ames, Iowa. Testing can take up to several weeks to complete depending upon the type of sample submitted and the testing protocol required to obtain a definitive result. Details on the diagnostic criteria for EEE for can be found at: https://www.aphis.usda.gov/aphis/ourfocus/animalhealth/animal-disease-information/equine/eee-wee-vee/equine-encephalitis
For additional details, see the section, Laboratory Diagnosis of Human Arboviral Diseases.
Virus Detection
Methods for virus detection, isolation, and identification are the same as described for human and mosquito diagnostics. The most commonly used methods to detect EEE virus or viral RNA in animal populations are immunoassays, virus isolation, and molecular tests. Specimens typically are tissues or fluids from acutely ill or dead animals. Virus detection in apparently healthy animals is very low-yield and inefficient, and therefore not cost-effective, and should not be considered for routine surveillance programs. Some animals have few tissues with detectable virus particles or viral RNA at necropsy, such as horses. Others, such as certain bird species, may have fulminant infections with high viral loads in almost every tissue.
Integrated Vector Management
Prevention and control of arboviral diseases is accomplished most effectively through a comprehensive, Integrated Vector Management (IVM) program applying the principles of Integrated Pest Management. IVM is based on an understanding of the underlying biology of the arbovirus transmission system and utilizes regular monitoring of vector mosquito populations and arboviral activity levels to determine if, when, and where interventions are needed to keep mosquito numbers below levels which produce risk of human disease, and to respond appropriately to reduce risk when it exceeds acceptable levels.
Operationally, IVM is anchored by a monitoring program providing data that describe
- Conditions and habitats that produce vector mosquitoes
- Abundance of those mosquitoes over the course of a season
- Arboviral transmission activity levels expressed as infection rate in mosquito vectors
- Parameters that influence local mosquito populations and virus transmission
These data inform decisions about implementing mosquito control activities appropriate to the situation, such as
- Source reduction through habitat modification
- Larval mosquito control using the appropriate methods for the habitat
- Adult mosquito control using pesticides applied from trucks or aircraft when established thresholds have been exceeded
- Community education efforts related to risk levels and intervention activities
Monitoring also provides quality control for the program, allowing evaluation of the effectiveness of larval and adult control efforts, and causes of control failures (e.g., undetected larval sources, pesticide resistance, equipment failure).
Mosquito Control Activities
Mosquito control tools target mosquitoes at the adult or immature stage depending on program objectives. Multiple species are involved in eastern equine encephalitis (EEE) virus transmission, and different populations of a single species may vary their activity based on environmental conditions. The decision to conduct mosquito control activities is based on mosquito and meteorological surveillance, established local thresholds and triggers (mosquito, human, and non-human animal), and insecticide resistance status of the target species (Table 1). Mosquito control professionals should have detailed knowledge of the local mosquitoes involved in EEE virus transmission to prevent and control disease. Programs should use pesticides and other control tools registered by the U.S. Environmental Protection Agency (EPA) in compliance with label instructions and any local, state, and federal laws regulating their use.
http://www.epa.gov/mosquitocontrol
Larval Mosquito Control
The objective of larval mosquito control is to reduce immature mosquito populations before they emerge as adults. This can be an efficient method of managing mosquitoes where larval sites are accessible, but habitats of EEE virus vectors are often hard to find and labor-intensive to treat. Few studies have shown efficacy of larval control methods against EEE virus vectors.
Culiseta melanura larvae develop in crypts filled with water in swamp and bog habitats. A single study showed aerial application of methoprene penetrated larval crypts and had 81% efficacy (emergence inhibition) over 5-weeks post-treatment (Woodrow et al. 1995). Temephos was also evaluated and not detected in the larval habitats (crypts) of Cs. melanura. Although not evaluated yet for EEE, aerial or ULV Bacillus thuringiensis israelensis (Bti) water-dispersible granules can penetrate foliage and water in covered areas to control other mosquitoes that occur in cryptic larval habitats (e.g., Aedes aegypti, Culex quinquefasciatus) (Pruszinski et al. 2017). These delivery techniques may be also useful against Cs. melanura and the larval habitats of epizootic bridge vectors. Although further studies are needed on the efficacy and implementation of larval control of EEE virus, applying a larvicide at the same time as an adulticide application to reduce adult mosquito populations may help prevent population rebound due to newly emerged adults and mosquitoes not active at the time of application.
Larvicides (and pupacides) are applied directly to water sources or placed in areas where flooding is expected to target the aquatic habitats of vector species. Larvicide can be applied by ground or aerial dispersal methods. For small aquatic larval sites or areas that cannot be reached by vehicles, backpack sprayers and dusters are used to apply liquid, granules, or pellets. Formulations can be short-acting (up to 2 weeks) or extended-release products (lasting more than 1 month). Larvicides may kill on contact through ingestion, or act as stomach poisons or growth regulators. Information on pesticides for larval mosquito control is available from the U.S. EPA (http://www.epa.gov/mosquitocontrol/controlling-mosquitoes-larval-stage).
Adult Mosquito Control
Adult mosquito control aims to reduce the abundance of biting, infected adult mosquitoes to prevent them from transmitting arboviruses to humans and to break the mosquito-host transmission cycle. Where populations are increasing above acceptable levels, adulticides are used to reduce vectors. Vector mitigation strategies should be applied quickly once arboviral activity is detected and be targeted to the local EEE virus epizootic and enzootic vectors. Programs should use pesticides registered by EPA for this purpose (http://www.epa.gov/mosquitocontrol/controlling-adult-mosquitoes).
Adulticides can reduce the numbers of adult mosquito vectors for EEE virus, but not enough cases occur annually to demonstrate clear impact on EEE virus transmission to humans. Indicators of high transmission risk are used to decide when to apply adulticides and often by the time aerial applications occur, transmission to humans has already occurred. Also, due to the epidemic nature of this disease, untreated areas relevant for comparison might not be available, which limits the ability to make conclusions about the efficacy of using adulticides to reduce disease (Grady et al. 1978).
Adulticiding can be conducted from the ground with backpack spray equipment, truck-mounted equipment, or by air with fixed-wing or rotary-wing applications. Types of treatment include space-spray (e.g., ULV) adulticides and residual treatments.
- Space-spray and ULV treatments rely on mosquitoes and insecticide droplets coming into direct contact in the air column. These are temporary measures to reduce the mosquito population active at the time of treatment (Lloyd et al. 2018). ULV formulations applied in small volumes prevent deposition and enhance degradation of the active ingredients in the environment (Bonds 2012). Mosquitoes not active at the time of application are not exposed. Because there is little to no deposition of insecticide, no residual control of mosquitoes occurs. As a result, multiple applications may be needed for sustained control (Andis et al. 1987).
- Long-lasting adulticides, also called residual or barrier treatments, can be applied to surfaces and to vegetation. To be effective, the mosquito must land on the treated surface and directly contact the insecticide. This type of application targets the resting mosquito population and is typically used in urban pest management and residential properties (Lloyd et al. 2018).
- Other methods of control: Traps and baits have been proposed to control mosquitoes (e.g., Ae. aegypti, Ae. albopictus) but few studies have been conducted for vectors of EEE virus. A single study on attractive targeted sugar baits (ATSB), which attract and kill sugar feeding mosquitoes, found reductions in adult Cs. melanura populations in the 2-weeks post-treatment; however, the study design, number of mosquitoes trapped, and background insecticide used in the study limit the conclusions. At present, more evidence is needed before for broad scale use can be recommended. (Qualls et al. 2014).
Safety and Quality of Vector Control Pesticides and Practices
Insecticides to control larval and adult mosquitoes are registered specifically for that use by the EPA. Instructions provided on the product labels prescribe the required application and use parameters and must be carefully followed. Properly applied, these products do not negatively affect human health or the environment. In persons living in treated areas, ULV application of mosquito control adulticides does not produce any detectable biological changes indicating exposure or increase asthma or other adverse health events (Currier et al. 2005; Duprey et al. 2008; Karpati et al. 2004). The morbidity and mortality from arboviruses demonstrably exceed the risks from mosquito control practices (Davis and Peterson 2008; Macedo et al. 2010; Peterson et al. 2006).
Legal Action to Achieve Access or Control
Individually owned private properties may be major sources of mosquito production. Examples include accumulations of discarded tires or other trash, neglected swimming pools, and similar water features that become stagnant and produce mosquitoes. Local public health statutes or public nuisance regulations may be employed to gain access for surveillance and control or to require the property owner to mitigate the problem. Executing such legal actions may be a prolonged process during which adult mosquitoes are continuously produced. Proactive communication with residents and public education programs may alleviate the need to use legal actions. However, legal efforts may be required to eliminate persistent mosquito production sites.
Quality of Control
Pesticide products and application procedures (for both larval and adult control) must periodically be evaluated to ensure an effective rate of application is being used and that the desired degree of control is obtained. Application procedures should be evaluated regularly (minimally once each season) to assure equipment is functioning properly to deliver the correct dosages and droplet parameters and to determine appropriate label rates to use locally. Finally, mosquito populations should routinely be evaluated to ensure insecticide resistance is not emerging.
Records
Surveillance data describing vector sources, abundance and infection rates, records of control efforts (e.g., source reduction, larvicide applications, adulticide applications), and quality control data must be maintained and used to evaluate IVM needs and performance. Long-term data are essential to track trends and to evaluate levels of risk.
Insecticide Resistance Management
For vector control to be effective, mosquitoes must be susceptible to the insecticide selected for use. In order to delay or prevent the development of insecticide resistance in vector populations, IVM programs should include a resistance management component (Lloyd et al. 2018). This should include routine monitoring of the status of resistance in the target populations to
- Provide baseline data for program planning and pesticide selection before the start of control operations
- Detect resistance at an early stage so that timely management can be implemented
- Continuously monitor the effect of control strategies on insecticide resistance, and determine potential causes for control failures, should they occur
Insecticide resistance may be monitored using bioassays in larvae or adult mosquitoes (Brogden and McAllister 1998). The CDC bottle bioassay is a simple, rapid, and economical tool to detect insecticide resistance by determining the time taken for a pesticide active ingredient to kill mosquito vectors. The results can help guide the choice of insecticide used for spraying. The CDC bottle bioassay can be used as part of a broader insecticide resistance monitoring program, which may include field cage tests and biochemical and molecular methods. Download a practical laboratory manual for the CDC bottle bioassay. For additional information, contact CDC at [email protected].
The IVM program should include options for managing resistance that are appropriate for local conditions. The techniques regularly used include the following:
- Management by moderation. Prevent onset of insecticide resistance by reducing overall chemical use or persistence by
- Using doses no lower than the lowest label rate to avoid genetic selection
- Using chemicals of short environmental persistence and avoiding slow-release formulations that increase selection for resistance
- Avoiding use of the same class of insecticide to control adult and immature stages
- Applying locally;many districts treat only hot spots and use area-wide treatments only during public health alerts or outbreaks
- Using less frequent applications; leaving generations, population segments,or areas untreated (when appropriate)
- Establishing higher thresholds for mosquito mitigation with insecticides, except during public health alerts or outbreaks.
- Using doses no lower than the lowest label rate to avoid genetic selection
- Management by continued suppression. This strategy is used in regions of high value or persistent high risk (e.g., heavily populated regions or locations with recurring outbreaks) where mosquitoes must be kept at very low densities. It involves the application of dosages within label rates but sufficiently high to be lethal to heterozygous individuals that are partially resistant. If the heterozygous individuals are killed, resistance will be slow to emerge. This method should not be used if any significant portion of the population in question is fully resistant. Another approach more commonly used is the addition of synergists that inhibit existing detoxification enzymes and thus eliminate the competitive advantage of these individuals. Commonly, the synergist of choice in mosquito control is piperonyl butoxide (PBO).
- Management by multiple methodology. This strategy involves the use of insecticides with different modes of action in mixtures or in rotations. There are economic limitations associated with this approach (e.g., costs and logistics of switching or storing chemicals), and critical variables in addition to the pesticide mode of action that must be taken into consideration (i.e., mode of resistance inheritance, frequency of mutations, population dynamics of the target species, availability of refuges, and migration). Programs should evaluate resistance patterns routinely and the need for rotating insecticides at annual or longer intervals.
Continuing Education
Continuing education for operational vector control workers is required to instill or refresh knowledge related to practical mosquito control. Training focusses on safety, applied technology, and requirements for the regulated certification program mandated by most states. Training should also include information on the identification of mosquito species, their behavior, ecology, and appropriate methods of control.
Guidelines for a Phased Response
The objective of a phased response to EEE surveillance data is to implement public health interventions appropriate to the level of risk in a community (Table 2). A surveillance program adequate to monitor EEE virus activity levels associated with human risk must be in place to detect epizootic transmission in advance of human disease outbreaks. Human case reports lag behind human infection events and are poor indicators of current risk levels. Effective public health action depends on interpreting the best available surveillance data and initiating prompt and aggressive intervention when necessary.
Individual-Level Actions to Reduce Risk
Without an effective vaccine for people, the best way to prevent mosquito-borne disease is by preventing mosquito bites. This can be accomplished through community-based IVM programs and by personal protection behaviors, such as
- Mosquito-avoidance. Health officials may recommend residents avoid outdoor activities when high virus activity levels have been detected or when mosquitoes are most active.
- Use of personal insect repellents. CDC recommends using EPA-registered insect repellents or covering up with long-sleeved shirts and long pants when outside.
- Removal of residential mosquito sources. Once a week, residents should empty, cover, or throw out items that hold water, such as tires, buckets, planters, toys, pools, birdbaths, flowerpots, or trash containers.
Jurisdictions can promote individual and community-based prevention measures through public education and risk communication activities. Messages should acknowledge the seriousness of the disease without promoting undue fear or panic in the target population. Fear-driven messages may heighten the powerlessness people express in dealing with vector-borne diseases. Messages should be clear and consistent with the recommendations of coordinating agencies and include a call to action. Use plain language and adapt materials for lower literacy and non-English speaking audiences.
People can further reduce their risk of mosquito bites by not going outdoors when mosquitoes are biting and following recommendations to avoid outdoor activity when and where high virus activity levels have been detected are a component of prevention programs. Recommendations to avoid being outdoors during peak mosquito biting times may conflict with neighborhood social patterns, community events, people's jobs or the practices of persons without air-conditioning. It is important to communicate when the important mosquito vectors are most active. Emphasize that insect repellent use is protective and should be used when outdoors, particularly during the prime mosquito-biting hours.
Additional information about personal insect repellents, including permethrin, can be found at the CDC Mosquitoes website.
Information for individuals on control mosquitoes around their home can be found at the CDC Mosquitoes website.
Prevention Strategies for High-Risk Groups
Audience members have different disease-related concerns and motivations for action. Proper message targeting (including use of plain language) permits better use of limited communication and prevention resources. The following are some population segments that require specific targeting. See Human Disease Section for additional information about risk groups that should be targeted.
- Persons with Outdoor Exposure. People who engage in extensive outdoor work or recreational activities are at greater risk of being bitten by mosquitoes. Messages for these individuals should encourage use of insect repellent and long-sleeved shirts and pants. Local spokespersons (e.g., union officials, job-site supervisors, golf pros, sports organizations, lawn care professionals, public works officials, gardening experts) may be useful collaborators. Place messages in locations where people engage in outdoor activities (e.g., parks, golf courses, hiking trails).
- People Experiencing Homelessness. Extensive outdoor exposure and limited financial resources in this group present special challenges. Application of insect repellents to exposed skin and clothing may be most appropriate prevention measures for this population. Work with social service groups in your area to educate and provide insect repellents to this population segment.
- Residences Lacking Window and Door Screens. The absence of intact window/door screens might increase exposure to mosquito bites. Encourage residents to consistently use screened windows and doors to keep mosquitoes outside. Focus attention on the need to repair screens and provide access to resources to do so. Partner with community organizations that can assist with installing or repairing screens for older persons or others with financial or physical barriers.
- Older Adults. For many mosquito-borne diseases, older adults are at greater risk for serious disease. Messages on mosquito avoidance, insect repellent use, and weekly removal of standing water where mosquitoes lay eggs around the home should be shared with this audience.
Communication And Community Engagement
At the community level, advocating for organized mosquito abatement and participating in community mobilization projects to address sources of mosquitoes such as trash, standing water, or untreated swimming pools are activities that can help protect individuals and at-risk groups.
Providing clear messages and understandable concepts promotes community understanding and acceptance. The following provides a description of selected best practices for reaching high-risk groups, offers suggestions for cultivating partnerships with media and communities, and provides select outreach measures for mobilizing communities.
- Communicating about Vector Control. Public understanding and acceptance of emergency adult mosquito control operations using insecticides is critical to its success, especially where these measures are unfamiliar. Questions about the products being used, their safety, and their effects on the environment are common. Improved communication about surveillance and how decisions to use mosquito adulticides are made may help residents weigh the risks and benefits of control. When possible, provide detailed information regarding the schedule for adulticiding through newspapers, radio, government-access television, the internet, recorded phone messages, social media, or other means your agency uses to successfully communicate with its constituencies.
- Community Mobilization and Outreach. Community mobilization can improve education and help achieve behavior change goals. Promote the concept that health departments and mosquito control programs require community assistance to reduce mosquito-borne disease risk. Leverage online platforms to further disseminate your messages.
- A community task force that addresses civic, business, public health, and environmental concerns can be valuable in achieving buy-in from various segments of the community, and in developing common messages. Community mobilization activities can include clean-up days to get rid of mosquito habitats (e.g., tires, trash). Effective community outreach also involves presenting messages in person, involving citizens in prevention and control activities, and using traditional and social media outreach. Hearing the message of personal prevention from community leaders can validate the importance of the disease and serve as a community call to action. Health promotion events and activities reinforce the importance of prevention and control in a community setting.
- A community task force that addresses civic, business, public health, and environmental concerns can be valuable in achieving buy-in from various segments of the community, and in developing common messages. Community mobilization activities can include clean-up days to get rid of mosquito habitats (e.g., tires, trash). Effective community outreach also involves presenting messages in person, involving citizens in prevention and control activities, and using traditional and social media outreach. Hearing the message of personal prevention from community leaders can validate the importance of the disease and serve as a community call to action. Health promotion events and activities reinforce the importance of prevention and control in a community setting.
- Partnership with Media and the Community. It can be beneficial to cultivate relationships with the media (e.g., radio, TV, newspaper, web-based news outlets) prior to an outbreak. Obtain media training for at least one member of your staff and designate that individual as the organization's spokesperson. Develop key messages and a communication plan, including press releases, prior to developing products. Many communities have heard mosquito prevention and control messages repeated for several years. Getting the public's attention when risk levels increase can be a challenge. Therefore, evaluate and update mosquito bite prevention messages annually and test new messages with different population segments to evaluate effectiveness. Develop partnerships with agencies and organizations that have relationships with populations at higher risk (such as persons over 50 years of age) or are recognized as community leaders (e.g., churches, service groups). Working through sources trusted by the priority audience can heighten the credibility of and attention to messages. Partnerships with businesses that sell materials to fix or install window screens or that sell insect repellent may be useful in some settings (e.g., local hardware stores, grocery stores).
- Social Media. A majority of Americans use social media which can be an inexpensive and rapid method for disseminating information to the community. Outreach can be conducted using Twitter, Facebook, YouTube, blogs, and other websites that may reach constituents less connected to more traditional media sources. Using images or videos in your posts make them more attention grabbing. It is also best practice to include a call-to-action people can take. Provide links that direct users to webpages or other resources with more complete information.
- Online Resources. The Internet has become a primary source of health information for most Americans. Encourage constituents to seek advice from credible sources. Make sure local public health agency websites are clear, accurate, and up to date. Useful information is available from a number of resources:
- The CDC web pages are updated frequently to reflect new findings and recommendations. Materials on the CDC web site are in the public domain and serve as a resource for state and local health departments and other organizations.
- CDC staff can provide technical assistance in the development of audience research and strategies for public education and community outreach. Contact CDC, Division of Vector-Borne Diseases' health communications staff in Fort Collins, CO at 970-221-6400.
- The U.S. Environmental Protection Agency (EPA) is the government's regulatory agency for insecticide and insect repellent use, safety, and effectiveness. Information about mosquito control insecticides and repellents is available at https://www.epa.gov/mosquitocontrol. These include guidance for using insect repellents safely and a search tool to assist in finding a repellent that is right for you (https://www.epa.gov/insect-repellents/find-repellent-right-you#searchform), which allows the user to examine the protection time afforded by registered insect repellents containing various concentrations of the active ingredients.
- There are several non-governmental organizations that have developed useful tools and information that can be adapted for local needs. Examples include: the American Mosquito Control Association (https://www.mosquito.org/default.aspx) and the National Pesticide Information Center (NPIC) (www.npic.orst.edu).
- There are several non-governmental organizations that have developed useful tools and information that can be adapted for local needs. Examples include: the American Mosquito Control Association (https://www.mosquito.org/default.aspx) and the National Pesticide Information Center (NPIC) (www.npic.orst.edu).
- The CDC web pages are updated frequently to reflect new findings and recommendations. Materials on the CDC web site are in the public domain and serve as a resource for state and local health departments and other organizations.
Before conducting any EEE virus testing, note:
EEE virus is an HHS Select Agent, and therefore, subject to strict regulations regarding its possession and use. Those intending to conduct EEE virus testing must be familiar with the complete information and specific guidance found at the Federal Select Agent Program website before conducting EEE virus testing.
Briefly, samples determined to be positive for EEE virus must be documented and reported to the Federal Select Agent Program via Form 4 (https://www.selectagents.gov/form4.html) within 7 calendar days of identification, and, if not diagnosed at a registered entity, they must then be transferred to a registered Select Agent facility or destroyed.
Testing Algorithm. All samples are screened for virus using either or both sets of the primers/probes listed below. A positive result in any of the negative controls invalidates the entire run. Failure of the positive control to generate a positive result also invalidates the entire run. A sample that is positive with one primer set and negative with the second set is classified as equivocal.
Note: At the CDC, Division of Vector-borne Diseases, Arboviral Disease Branch, the kits and protocols used by the Entomology and Ecology team are described below; however, there are several other options for RNA extraction and real-time RT-PCR on the market.
Results Interpretation
We use the following algorithm to evaluate the results.
Positive: Ct value ≤ 37
Negative: Ct value > 37
PCR PLATE SET-UP:
- Prepare primers and probes according to the following concentrations:
- Real-time RT-PCR master mix should be prepared in a "clean room" physically separated from all other laboratory activities with dedicated reagents and equipment (i.e., pipettes). Combine the reagents listed below in an RNase free centrifuge tube on ice. Using Qiagen's Quantitect Probe RT-PCR kit (#204443), prepare master mix as follows:Per reaction:
- 0 µl master-mix
- 2 µl water* (nuclease-free)
- 5 µl 100µM forward primer
- 5 µl 100µM reverse primer
- 3 µl 25µM probe
- 5 µl RT enzymeAdd about 5-10 reactions to your total number of samples (and account for "No template controls" (NTCs), positive controls, and negative extraction controls) and multiply number by volumes above.Example: You have 20 samples (12 unknown samples, 2 positive controls, 2 negative controls, and 4 NTCs). Make a master mix for 25 to 30 samples.
- NTC = mix ONLY with no sample, to test mix components (PCR control)
- Negative control = extracted water (extraction control)
- Pipette 45 µl of master mix* into either 0.2 ml optical (specifically for real-time assays; emission fluorescence is read through the cap) PCR tubes or a 96-well optical PCR plate. Use a reservoir and a multichannel pipette for many wells.
- Pipette 5 µl of RNA* into each well. Refer to a template to ensure that the proper sample is added to the corresponding well. Do not add anything to NTC samples (master mix only).
*The volume of RNA added per reaction is typically 5 µl but can be increased (up to 25 µl) with the appropriate adjustment of the water in the master mix. For example, if you want to test 10 µl RNA, reduce the water per reaction to 13.2 µl, and add 40 µl master mix and 10 µl RNA to each well.
Cycling conditions (QIAGEN conditions for Real Time RT-PCR):
1 cycle each:
50°C for 30 min
95°C for 15 min
45 cycles:
95°C for 15 sec
60°C for 1 min (data collection step)
EEEV primers and probes. There are one published and one unpublished primer/probe sets available for the detection of EEEV RNA.
Published: Lambert et al. 2003.
EEEV 9391 F ACACCGCACCCTGATTTTACA
EEEV 9459 R CTTCCAAGTGACCTGGTCGTC
EEEV 9414-probe TGCACCCGGACCATCCGACCT
(unpublished)
EEEV 1898 F ACCTTGCTGACGACCAGGTC
EEEV 1968 R GTTGTTGGTCGCTCAATCCA
EEEV 1919-probe CTTGGAAGTGATGCAAATCCACTCGACA
Rna Extraction Tips
NOTES: Avoid contamination while working with RNA
- Maintain physically separated work areas; one dedicated to pre-amplification RNA work (RNA extraction) and the other for master mix production.
- Utilize dedicated/separate equipment within pre and post amplification areas; especially pipettes and centrifuges.
- Always wear gloves; even when handling unopened tubes.
- Open and close tubes quickly and avoid touching any inside portion.
- Use RNase free plastic disposable tubes and pipet tips.
- Use aerosol block pipet tips.
- Use RNase free water.
- Prepare all reagents on ice.
- Solid phase samples (mosquitoes or tissues) are first homogenized in an isotonic buffer to produce a liquid homogenate. Mosquito specimens are homogenized using copper clad steel bead (BB) grinding technique using a vortexer or mixer mill (i.e., Qiagen Tissuelyser). Homogenates are clarified by centrifugation in a microcentrifuge (i.e., Eppendorf) at maximum speed for 5 minutes to pellet any particulate material.
- Extract RNA from the clarified supernatant using the QiaAmp viral RNA kit (QIAGEN part #52904) or another comparable kit specifically designed to purify RNA. Follow the manufacturer's protocol exactly with the following modification for mosquito specimens: include 1 additional wash/centrifugation step with AW1, if using the Qiagen kit. Extract at least two negative controls and two positive controls along with the test specimens. The positive controls should differ in the amount of target RNA present (i.e., a pre-determined high positive and a low positive). Note: The volume of sample extracted can be greater or less than the standard volume stated in the QIAGEN protocol (140 µl) with the appropriate adjustments to all other volumes in the protocol. CDC typically extracts 100 µl.
Andis MD, Sackett SR, Carroll MK, Bordes ES. Strategies for the emergency control of arboviral epidemics in New Orleans. J Am Mosq Control Assoc 1987;3:125–130.
Andreadis TG, Armstrong PM. A two-year evaluation of elevated canopy trapping for Culex mosquitoes and West Nile virus in an operational surveillance program in the Northeastern United States. J Am Mosq Control Assoc 2007;23:137–148.
Andreadis TG, Anderson JF, Vossbrinck CR, Main AJ. Epidemiology of West Nile virus in Connecticut, USA: A five-year analysis of mosquito data, 1999-2003. Vector Borne Zoonotic Dis 2004;4:360–378.
Andreadis TG, Anderson JF, Tirrell-Peck SJ. Multiple isolations of eastern equine encephalitis and Highlands J viruses from mosquitoes (Diptera: Culicidae) during a 1996 epizootic in southeastern Connecticut. J Med Entomol 1998;35:296–302.
Armstrong PM and Andreadis TG. Eastern equine encephalitis virus in mosquitoes and their role as bridge vectors. Emerg Infect Dis 2010;16(12):1869–74.
Armstrong PM and Andreadis TG. Ecology and epidemiology of eastern equine encephalitis virus in the Northeastern United States: An historical perspective. J Med Entomol 2022;59(1):1–13.
Armstrong PM, Andreadis TG, Finan SL, Shepard JJ, Thomas MC. Detection of infectious virus from field-collected mosquitoes by Vero cell culture assay. J Vis Exp 2011;pii2889.
Armstrong PM, Prince N, Andreadis TG. Development of a multi-target TaqMan assay to detect eastern equine encephalitis virus variants in mosquitoes. Vector Borne Zoonotic Dis 2012;12(10):872–876.
Ayres JC and Feemster RF. The sequelae of eastern equine encephalomyelitis. N Engl J Med 1949;240: 960–962.
Basile AJ, Horiuchi K, Panella AJ, Laven J, Kosoy O, Lanciotti RS, et al. Multiplex microsphere immunoassays for the detection of IgM and IgG to arboviral diseases. PLoS One 2013;8:e75670.
Bell JA, Mickelson NJ, Vaughan JA. West Nile virus in host-seeking mosquitoes within a residential neighborhood in Grand Forks, North Dakota. Vector-Borne Zoonotic Dis 2005;5:373.
Berl E, Eisen RJ, MacMillan K, Swope BN, Saxton-Shaw K‑D, Graham AC, et al. Serological evidence for eastern equine encephalitis virus activity in white-tailed deer, Odocoileus virginianus, in Vermont, 2010. Am J Trop Med Hyg 2013;88:103–107.
Bigler WJ, Lassing E, Buff E, Lewis AL, Hoff GL. Arbovirus surveillance in Florida: Wild vertebrate studies 1965-1974. J Wildl Dis 1975;11:348–356.
Bolling BG, Barker CM, Moore CG, Pape WJ, Eisen L. Seasonal patterns for entomological measures of risk for exposure to Culex vectors and West Nile virus in relation to human disease cases in Northeastern Colorado. J Med Entomol 2009;46:1519–1531.
Bonds JA. Ultra-low-volume space sprays in mosquito control: a critical review. Med Vet Entomol 2012;26:121–130.
Brogdon WG, McAllister JC. Insecticide resistance and vector control. Emerg Infect Dis 1998;4:605–613.
Burkett-Cadena ND, Day JF, Unnasch TR. Ecology of eastern equine encephalitis virus in the Southeastern United States: Incriminating vector and host species responsible for virus amplification, persistence, and dispersal. J Med Entomol 2022;59(1):41–48.
Calisher CH. Medically important arboviruses of the United States and Canada. Clin Microbiol Rev 1994;7:89–116.
Callahan JD, Wu SJ, Dion-Schultz A, Mangold BE, Peruski LF, Watts DM, et al.. Development and evaluation of serotype- and group-specific fluorogenic reverse transcriptase PCR (TaqMan) assays for dengue virus. J Clin Microbiol 2001;39:4119–4124.
CDC. Data and Maps for Eastern Equine Encephalitis. Fort Collins; 2021a.
CDC. Clinical Signs and Symptoms of Eastern Equine Encephalitis. Fort Collins; 2021b.
CDC. Eastern equine encephalitis–New Hampshire and Massachusetts, August–September 2005. Morb Mortal Wkly Rep 2006;55(25):697–700.
Clarke DH. Two nonfatal human infections with the virus of eastern encephalitis. Am J Trop Med Hyg 1961;10:67–70.
Colborn JM, Smith KA, Townsend J, Damian D, Nasci RS, Mutebi JP. West Nile virus outbreak in Phoenix, Arizona—2010: Entomological observations and epidemiological correlations. J Amer Mosq Control Assoc 2013;29:123–32.
Crans WJ, Caccamise DF, McNelly JR. Eastern equine encephalomyelitis virus in relation to the avian community of a coastal cedar swamp. J Med Entomol 1994;31:711–728.
Crans WJ. Failure of chickens to act as sentinels during an epizootic of eastern equine encephalitis in southern New Jersey, USA. J Med Entomol 1986;23(6):626–9.
Crans WJ. The status of Aedes sollicitans as an epidemic vector of eastern equine encephalitis in New Jersey. Mosq News. 1977;37(1):85–9.
Currier M, McNeill, M, Campbell D, Newton N, Marr JS, Perry E et al. Human exposure to mosquito-control pesticides—Mississippi, North Carolina, and Virginia, 2002 and 2003. Morb Mortal Wkly Rep 2005;54:529–532.
Dalrymple JM, Young OP, Eldridge BF, Russell PK. Ecology of arboviruses in a Maryland freshwater swamp. III. Vertebrate hosts. Am J Epidemiol 1972;96:129–140.
Davis LE, Beckham JD, Tyler KL. North American encephalitic arboviruses. Neurol Clin 2008;26:727–57, ix.
Davis RS, Peterson RK. 2008. Effects of single and multiple applications of mosquito insecticides on nontarget arthropods. J Am Mosq Control Assoc 2008;24:270–280.
DeNicola AJ, VerCauteren KC, Curtis PD, Hygnstrom SE. Managing white-tailed deer in suburban environments: A technical guide. A publication of Cornell Cooperative Extension, the Wildlife Society—Wildlife Management Working Group, and Northeastern Wildlife Damage Research and Outreach Cooperative. New York (NY). 2000.
Deresiewicz RL, Thaler SJ, Hsu L, Zamani AA. Clinical and neuroradiographic manifestations of eastern equine encephalitis. N Engl J Med 1997;336:1867–1874.
Duprey Z, Rivers S, Luber G, Becker A, Blackmore C, Barr D, et al. Community aerial mosquito control and naled exposure. J Am Mosq Control Assoc 2008;24:42–46.
Eldridge BF. Surveillance for arthropodborne diseases. J Med Entomol. Eldridge BF, Edman JD (eds). Dordrecht (The Netherlands): Kluwer Academic Press; 2004. p. 645.
Elias SP, Keenan P, Kenney JL, Morris SR, Covino KM, Robinson S, et al. Seasonal patterns in eastern equine encephalitis
virus antibody in songbirds in southern Maine. Vector Borne Zoonotic Dis 2017;17:325–330.
Emord DE, Morris CD. Epizootiology of eastern equine encephalomyelitis virus in upstate New York, USA. VI. Antibody prevalence in wild birds during an interepizootic period. J Med Entomol 1984;21:395–404.
Feemster RF. Outbreak of encephalitis in man due to the eastern virus of equine encephalomyelitis. Am J Public Health Nations Health 1938;28:1403–1410.
Florida Coordinating Council on Mosquito Control. Florida Mosquito Control: The state of the mission as defined by mosquito controllers, regulators, and environmental managers. Lloyd AM, Connelly CR, and Carlson DB (Eds.). Vero Beach (FL): University of Florida, Institute of Food and Agricultural Sciences, Florida Medical Entomology Laboratory; 2018.
Gaensbauer JT, Lindsey NP, Messacar K, Staples JE, Fischer M. Neuroinvasive arboviral disease in the United States: 2003 to 2012. Pediatrics 2014;134:e642–e650.
Godsey MS Jr, Burkhalter K, Young G, Delorey M, Smith K, Townsend J, et al. Entomologic investigations during an outbreak of West Nile virus disease in Maricopa County, Arizona, 2010. Am J Trop Med Hyg 2012;87:1125–1131.
Goldfield M and Sussman O. The 1959 outbreak of eastern encephalitis in New Jersey. I. Introduction and description of outbreak. Am J Epidemiol 1968;87:1–10.
Goldfield M, Welsh JN, Taylor BF. The 1959 outbreak of eastern encephalitis in New Jersey. The inapparent infection: Disease ratio. Am J Epidemiol 1968;87:32–38.
Goodman CH, Russell BJ, Velez JO, Laven JJ, Bagarozzi DA Jr, Moon JL, et al. Production of a Sindbis/eastern equine encephalitis chimeric virus inactivated cell culture antigen. J Virol Methods 2015;223:19–24.
Grady GF, Maxfield HK, Hildreth SW, Timperi RJ Jr, Gilfillan RF, Rosenau BJ, et al. Eastern equine encephalitis in Massachusetts, 1957-1976: A prospective study centered upon analyses of mosquitoes. Am J Epidemiol 1978;107:170–178.
Graham SP, Hassan HK, Chapman T, White G, Guyer C, Unnasch TR. Serosurveillance of eastern equine encephalitis virus in amphibians and reptiles from Alabama, USA. Am J Trop Med Hyg 2012;86(3):540.
Gu W, Unnasch TR, Katholi CR, Lampman R, Novak RJ. Fundamental issues in mosquito surveillance for arboviral transmission. Trans R Soc Trop Med Hyg 2008;102:817–822.
Gujral IB, Zielinski-Gutierrez EC, LeBailly A, Nasci R. Behavioral risks for West Nile virus disease, northern Colorado, 2003. Emerg Infect Dis 2007;13:419–425.
Hayes RO, Beadle LD, Hess AD, Sussman O, Bonese MJ. Entomological aspects of the 1959 outbreak of eastern encephalitis in New Jersey. Am J Trop Med Hyg 1962;11:115–121.
Hoff GL, Issel CJ, Trainer DO, Richards SH. Arbovirus serology in North Dakota Mule and White-Tailed Deer. J Wildl Dis 1973; 9:291–295.
Holderman CJ, Gezan SA, Stone AES, Connelly CR, Kaufman PE. Mosquitoes (Diptera: Culicidae) collected from residential yards and dog kennels in Florida using two aspirators, a sweep net, or a CDC trap. J Med Entomol 2018;55(1):230-6.
Jones RC, Weaver KN, Smith S, Blanco C, Flores C, Gibbs K, et al. Use of the vector index and geographic information system to prospectively inform West Nile virus interventions. J Am Mosq Control Assoc 2011;27:315–319.
Karpati AM, Perrin MC, Matte T, Leighton J, Schwartz J, Barr RG. Pesticide spraying for West Nile virus control and emergency department asthma visits in New York City, 2000. Environ Health Perspect 2004;112:1183–1187.
Kwan JL, Park BK, Carpenter TE, Ngo V, Civen R, Reisen WK. Comparison of enzootic risk measures for predicting West Nile disease, Los Angeles, California, USA, 2004-2010. Emerg Infect Dis 2012;18:1298–1306.
Lambert AJ, Martin DA, Lanciotti RS. Detection of North American eastern and western equine encephalitis viruses by nucleic acid amplification assays. J Clin Microbiol 2003 41:379–385.
Lanciotti RS, Kerst AJ, Nasci RS, Godsey MS, Mitchell CJ, Savage HM, et al. Rapid detection of West Nile virus from human clinical specimens, field-collected mosquitoes, and avian samples by a TaqMan reverse transcriptase-PCR assay. J Clin Microbiol 2000;38:4066–4071.
Letson GW, Bailey RE, Pearson J, Tsai TF. Eastern equine encephalitis (EEE): a description of the 1989 outbreak, recent epidemiologic trends, and the association of rainfall with EEE occurrence. Am J Trop Med Hyg 1993;49:677–85.
Lindsey NP, et al. Eastern equine encephalitis in the United States, 2003–2016. Am J Trop Med Hyg 2018;98:1472–1477.
Lothrop HD, Lothrop BB, Gomsi DE, Reisen WK. Intensive early season adulticide applications decrease arbovirus transmission throughout the Coachella Valley, Riverside County, California. Vector Borne Zoonotic Dis 2008;8:475–490.
Lubelczyk C, Elias SP, Kantar L, Albert J, Hansen S, Saxton-Shaw K, et al. Detection of eastern equine encephalitis virus antibodies in moose (Alces americana), Maine, 2010. Vector Borne Zoonotic Dis 2014; 14:77–81.
Macedo PA, Schleier JJ 3rd, Reed M, Kelley K, Goodman GW, Brown DA, et al. Evaluation of efficacy and human health risk of aerial ultra-low volume applications of pyrethrins and piperonyl butoxide for adult mosquito management in response to West Nile virus activity in Sacramento County, California. J Am Mosq Control Assoc 2010;26:57–66.
Marchinton RL, Hirth DH. Behavior in White-tailed deer: Ecology and management. Halls LK (ed). Wildlife Management Institute: Stackpole Books; 1984. p. 129–168.
McCardle PW, Webb RE, Norden BB, Aldrich JR. Evaluation of five trapping systems for the surveillance of gravid mosquitoes in Prince Georges County, Maryland. J Am Mosq Control Assoc 2004;20:254–260.
McLean RG, Frier G, Parham GL, Francy DB, Monath TP, Campos EG, et al. Investigations of the vertebrate hosts of eastern equine encephalitis during an epizootic in Michigan, 1980. Am J Trop Med Hyg 1985;34:1190–1202.
Miley KM, Downs J, Burkett-Cadena ND, West RG, Hunt B, Deskins G, et al. Field analysis of biological factors associated with sites at high and low to moderate risk for eastern equine encephalitis virus winter activity in Florida. J Med Entomol 2021;8:2385–2397.
Molaei G, Armstrong PM, Graham AC, Kramer LD, Andreadis TG. Insights into the recent emergence and expansion of eastern equine encephalitis virus in a new focus in the Northern New England USA. Parasit Vectors 2015;8:516.
Morris CD. Eastern equine encephalitis. The arboviruses: Epidemiology and ecology, Vol. 3. Monath TP, ed. Boca Raton (FL): CRC Press; 1988. p. 1–20.
Mutebi JP, Godsey M, Smith RP Jr, Renell MR, Smith L, Robinson S, et al. Prevalence of eastern equine encephalitis virus antibodies among white-tailed deer populations in Maine. Vector Borne Zoonotic Dis 2015;15:210–214.
Mutebi JP, Lubelczyk C, Eisen R, Panella N, Macmillan K, Godsey M, et al. Using wild white-tailed deer to detect eastern equine encephalitis virus activity in Maine. Vector Borne Zoonotic Dis 2011;11:1403–1409.
Mutebi JP, Swope BN, Saxton-Shaw KD, Graham AC, Turmel JP, Berl E. Eastern equine encephalitis in moose (Alces americanus) in northeastern Vermont. J Wildl Dis 2012;48:1109–1112.
Nasci RS, Berry RL, Restifo RA, Parsons MA, Smith GC, Martin DA. Eastern equine encephalitis virus in Ohio during 1991. J Med Entomol 1993;30(1):217–22.
Nasci RS. A lightweight battery-powered aspirator for collecting resting mosquitoes in the field. Mosq News 1981;41:808–811.
Olson JG, Reeves WC, Emmons RW, Milby MM. Correlation of Culex tarsalis population indices with the incidence of St. Louis encephalitis and western equine encephalomyelitis in California. Am J Trop Med Hyg 1979;28:335–343.
Panella NA, Crockett RJ, Biggerstaff BJ, Komar N. The Centers for Disease Control and Prevention resting trap: a novel device for collecting resting mosquitoes. J Am Mosq Control Assoc 2011;27:323–325.
Peterson RK, Macedo PA, Davis RS. A human-health risk assessment for West Nile virus and insecticides used in mosquito management. Environ Health Perspect 2006;114:366–372.
Pouch SM, Katugaha SB, Shieh WJ, Annambhotla P, Walker WL, Basavaraju SV, et al. Transmission of eastern equine encephalitis virus from an organ donor to 3 transplant recipients. Clin Infect Dis 2019;69:450–458.
Pruszynski CA, Hribar LJ, Mickle R, Leal AL. A large scale biorational approach using Bacillus thuringiensis israelensis (Strain AM65-52) for managing Aedes aegypti populations to prevent dengue, chikungunya and Zika transmission. PLoS One 2017;12:e0170079.
Przelomski MM, O’Rourke E, Grady GF, Berardi VP, Markley HG. Eastern equine encephalitis in Massachusetts: A report of 16 cases, 1970–1984. Neurology 1988;38:736–739.
Reisen WK, Boyce K, Cummings RC, Delgado O, Gutierrez A, Meyer RP, et al. Comparative effectiveness of three adult mosquito sampling methods in habitats representative of four different biomes of California. J Med Entomol 1999;36:23–29.
Reiter P, Jakob WL, Francy DB, Mullenix JB. Evaluation of the CDC gravid trap for the surveillance of St. Louis encephalitis vectors in Memphis, Tennessee. J Am Mosq Control Assoc 1986;2:209–211.
Saxton-Shaw KD, Ledermann JP, Kenney JL, Berl E, Graham AC, Russo JM, et al. The first outbreak of eastern equine encephalitis in Vermont: Outbreak description and phylogenetic relationships of the virus isolate. PLoS One 2015;10:e0128712.
Schmitt SM, Cooley TM, Fitzgerald SD, Bolin SR, Lim A, Schaefer SM, et al. An outbreak of eastern equine encephalitis virus in free-ranging white-tailed deer in Michigan. J Wildl Dis 2007;43:635–644.
Sherwood JA, Oliver J. Eastern equine encephalitis incubation time periods of 5 and 8 days. Pediatr Infect Dis J 2015;34:459–60.
Silverman MA, Misasi J, Smole S, Feldman HA, Cohen AB, Santagata S, et al. Eastern equine encephalitis in children, Massachusetts and New Hampshire, USA, 1970–2010. Emerg Infect Dis 2013;19:194–201.
Skaff NK, Armstrong PM, Andreadis TG, Cheruvelil KS. Wetland characteristics linked to broad-scale patterns in Culiseta melanura abundance and eastern equine encephalitis virus infection. Parasit Vectors 2017;10:501.
Smith GC, Francy DB, Campos EG, Katona P, Calisher CH. Correlation between human cases and antibody prevalence in house sparrows during a focal outbreak of St. Louis encephalitis in Mississippi, 1979. Mosq News 1983;43:322–325.
Stamm DD. Arbovirus studies in birds in south Alabama, 1959-1960. Am J Epidemiol 1968;87:127137.
Stamm DD. Studies on the ecology of equine encephalomyelitis. Am J Pub Hlth 1958;48:328–335.
Sutherland GL, Nasci RS. Detection of West Nile virus in large pools of mosquitoes. J Am Mosq Control Assoc 2007;23:389–395.
Tate CM, Howerth EW, Stallknecht DE, Allison AB, Fischer JR, Mead DG. Eastern equine encephalitis in a free-ranging white-tailed deer (Odocoileus virginianus). J Wildl Dis 2005;41:241–245.
Tsachalidis E, Sokos CK, Birtsas PK, Pasikou NK. The Australian crow trap and the Larsen trap: Their capture success in Greece. Proceedings of the 2006 Naxos International Conference on Sustainable Management and Development of Mountainous and Island Areas. Vol. II. 2006; pp. 325–329.
Turell MJ, Spring AR, Miller MK, Cannon CE. Effect of holding conditions on the detection of West Nile viral RNA by reverse transcriptase-polymerase chain reaction from mosquito (Diptera: Culicidae) pools. J Med Entomol 2002;39:1–3.
Vahey GM, Mathis S, Martin SW, Gould CV, Staples JE, Lindsey NP. West Nile virus and other domestic nationally notifiable arboviral diseases–United States, 2019. Morb Mortal Wkly Rep 2021;70:1069–1074.
Woodrow RJ, Howard JJ, White DJ. Field trials with methoprene, temephos, and Bacillus thuringiensis serovar israelensis for the control of larval Culiseta melanura. J Am Mosq Control Assoc 1995;11:424–427.